Very-High-Speed SDH
TDM Links and Switches
One obvious
solution is to push current high-speed TDM networks to even higher
speeds. This solution works for fairly high speeds: current SDH
links operate at 10 Gb/s (OC-192), and could be pushed up to 40 Gb/s
(OC-768). However, the technology seems to already be approaching
its limits, as dictated by the maximum speed of current electronics.
Optical transmitters and receivers are currently limited to 10 Gb/s
speeds. Furthermore, in some cases where old fiber is installed in
the ground, polarization mode dispersion limits the bit rate to less
than 10 Gb/s, 40 Gb/s for long distances. i
Parallel Fibers,
Parallel Electrical Switches
Another
straightforward solution is to use parallel fibers between sites
connected by lower-speed electrical switches. This is indeed a good
idea in places with a rich optical infrastructure. However, for long
distances this is still a costly solution, since each of these
fibers requires its own set of optical amplifiers every 80–120 km.
Such equipment constitutes a large portion of the fiber cost, needs
to be managed, and considerably complicates the system. This
solution is not very scalable, as additional parallel fibers will
result in linear increases in the cost. In places where there is not
enough fiber, the high costs of laying more fiber in the ground and
legal complexities involved in getting the "right-of-way" permission
from land owners to install it are the major disadvantages of this
solution.
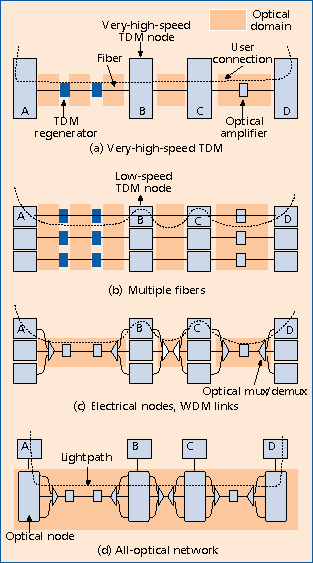
Figure 9 -
Alternatives for very high speed links i
WDM Links, Parallel
Electrical Switches
Here, the
parallel fibers of the previous solution are replaced by separate
channels of a single WDM link. These channels are interconnected by
lower-speed existing TDM equipment. An important cost advantage of
this solution over the previous one is that there is no need for an
amplifier per channel, and all the wavelengths are amplified
together by a single optical amplifier. A central advantage of
combining optical transmission with electrical switching over
all-optical networks (OTNs) is that it is based on today's
technology, and is thus cheaper, more reliable, and more flexible.
However, the
current deployment of point-to-point WDM technology supports little
in terms of networking functionality, and does not yet perform
traffic protection or restoration. In cases of fiber cuts or network
failures, synchronous digital hierarchy (SDH) equipment would
usually provide these functions, with WDM used strictly for fiber
capacity expansion. i
All-Optical Networks-OTN
Recent advances
in WDM technology -- WDM add/drop multiplexers (ADMs), optical
cross-connects (OXCs) -- with the ability to add, drop, and in
effect construct wavelength-switched and wavelength-routed networks,
are now beginning to shift the focus more toward optical networking
and network-level issues. As such, it presents an attractive
opportunity to evolve WDM technology toward an optical networking
infrastructure with transport, multiplexing, routing, supervision,
and survivability supported at the optical layer.
Wavelength
routing networks allow the setup of lightpaths which remain in the
optical domain across the network. Thus, they enable the creation of
configurable higher-level (logical) topologies based on traffic
analysis, and easy reconfiguration as traffic demands change. In any
case, it is clear that they offer an almost unlimited upgrade path
for the future, which is not the case with the other solutions.
i
Advantages
The all-optical
solution based on OTN has the following advantages over all the
other solutions:
-
Transparency
-
Future-Proofness
-
Reduced
Processing
-
Reduced
Management
Transparency
Since no
electrical processing is involved, wavelength routing networks are
not aware of the structure of the data, and can carry diverse
protocols and bit coding structures. Electrical solutions carry a
single form of traffic and require costly conversion devices from
other protocols to the supported standard, which also complicate the
management of the network. Another type of transparency supported by
wavelength routing networks (although to a lesser extent) is bit
rate transparency. Such networks will carry quite a large spectrum
of bit rates, up to a maximum rate determined by the design point of
the system.
Future-Proofness
A corollary of
the above is that all-optical networks will carry most future
protocols at many different bit rates without having to replace
components of the network. Thus, the investment in this technology
is protected against future developments.
Reduced Processing
Electrical
solutions involve considerably more processing than their
all-optical counterparts, a fact which implies more hardware or more
expensive hardware. Consider a bit in a connection between nodes A
and D in Fig 9-a-c. This bit is converted to the electrical domain,
re-clocked and processed by each and every node on the path (nodes B
and C in this example). In Fig 9d, however, there is a lightpath
from node A to node D, and our bit remains in the optical domain at
nodes B and C. Thus, the electrical switches of nodes B and C are
not bothered by it and can be made smaller, and less costly.
Reduced Management
Whenever a bit is
interpreted, an error may occur. In turn, this event must be
detected and reported (especially in the telco world, where network
management is much less oblivious to such events). Thus, if bits are
interpreted only at the border of a network, much less
quality-of-service-related management is necessary (fault management
is, however, still necessary).
Disadvantages
Disadvantages of
wavelength routing networks are the following.
Immaturity
At this stage,
optical components are not yet mature. Some of them are technically
mature -- for example, distributed feedback (DFB) lasers. Other
components are technically immature (e.g., optical switches), as
indicated by their large physical dimensions and their less reliable
nature. However, it seems that this is not an inherent problem and
will be resolved in the near future. i
System Design Problems
Many design
issues for wavelength routing network systems are not yet fully
understood and solved. Examples of such problems are the wavelength
allocation problem and the dynamic gain equalization problem. There
has been quite extensive research on wavelength allocation, but the
problem is far from being resolved efficiently, even for simple
network topologies. This fact limits the scalability of the network,
especially if the number of wavelengths per fiber is low.
A much more
severe obstacle for having scalable wavelength routing networks is
the physical layer design, particularly variation in the signal
quality of individual lightpaths, which is very hard to control.
Since optical amplifiers do not amplify all wavelengths by the same
amount, and some lightpaths travel many hops while others travel a
single hop, the energy of some lightpaths may be very low at their
destination, while others have high energy. Thus, it is necessary to
equalize the gain, for example, by having adaptive filters or
transmitting different energy levels depending on the route of
lightpaths. To further complicate the picture, the network has to
react to sudden changes in the configuration of lightpaths (e.g.,
due to link failures). This dynamic gain equalization problem is
very complex and far from being well understood or solved.i
State of ITU standards
As obvious from Figure 10, the
standards as regards to OTN are not ready yet, and most current
solutions are vendor dependent.
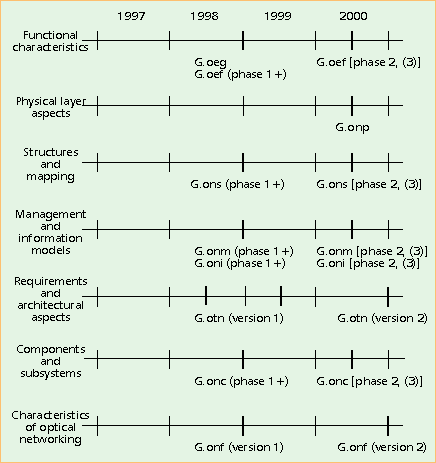
Figure 10 -
Expected time frame of ITU optical networking standards.
i
|