WDM Links
WDM technology is
based on the ability to transmit several light signals on a single
fiber using different wavelengths. It turns out that such different
light wavelengths do not interfere with each other, and thus can be
split apart at the other end of the fiber to form separate channels
(Fig.4 is a schematic drawing of such a system). In this figure only
half-duplex channels are depicted. To realize the other direction, a
duplicate system is typically used in that direction The WDM link
essentially comprises the following elements (scanning the figure
from left to right):
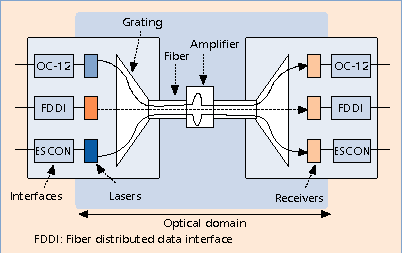
Figure 4 - A WDM
link.
-
·
Different interfaces
per port to enable different protocols to communicate over the
link.
-
·
An electro-optical
converter which includes a laser per channel at different
wavelengths.
-
·
An optical
multiplexer, typically a piece of glass called a grating.
-
·
Due to attenuation,
amplifiers may be needed along the fiber or at the endpoints.
-
·
When the signal gets
to the other end of the fiber it is split by an optical
demultiplexer, which acts like a prism, to separate
wavelength-specific optical signals (the same grating could be
used again).
-
·
A
wavelength-insensitive receiver converts the signal into
electrical form.
-
·
The signal is output
via the specific interface of the channel's port.
The pace of
improvement for this technology is spectacular: while in 1994 the
only commercial product for the telco market multiplexed four OC-48
(2.5 Gb/s) channels to a distance of 550 km, the high-end
multiplexer in 1995 has multiplexed 8 such channels, 16 channels to
600 km in 1996, and a 32-channel system to 1200 km in 1997, and much
more (100s) has been announced currently. As for the enterprise
market, a multi-protocol lower-speed and shorter-distance system has
existed since 1994. As mentioned earlier, such systems are already
deployed and are rapidly gaining popularity due to their maturity,
the large gain they offer, and the simplicity of integration with
legacy equipment.
Several other demonstrations of
WDM that have been reported recently are:
- 34 wavelengths x10 Gb/s signal
transmitted over 8 514 km fiber using an equalization technique
via Fourier synthesis of the gain characteristic of the system;
- 50 GHz spaced, 32 wavelengths
x10 Gb/s signal trans-mitted over 640 km dispersion-shifted
fiber with multi-wavelength distributed Raman amplification;
- 25 wavelengths x40 Gb/s, 1
Tb/s throughput experiment demonstrated over 342 km in nonzero
dispersion fiber.
Early deployment
of WDM has mainly been point-to-point to increase link bandwidth,
supports little in terms of networking functionality, and does not
yet perform traffic protection or restoration. In cases of fiber
cuts or network failures, synchronous optical network/synchronous
digital hierarchy (SONET/SDH) equipment would usually provide these
functions, with WDM used strictly for fiber capacity expansion.
WDM Link Enabling Technologies
The optical WDM
revolution in transmission and emerging applications in optical
networking are enabled by a range of key optical technologies. At
the foundation is the low loss, 0.25 dB/km, single-mode optical
fiber, which allows long-distance transmission with a bandwidth
window of about 25 THz.
Source category |
Tuning range |
Tuning time |
Comments |
Mechanically tuned
lasers |
Full range of laser
(1020 nm) |
100500 ms |
Mechanical tuning of
the facets of an FP external cavity |
Acousto-optically and
electro-optically tuned lasers |
Full range of laser
(1020 nm) |
Tens of s |
Find application in
packet switching |
Injection current
tuned lasers |
4 nm |
0.510 ns |
Limited tuning range |
Switched sources |
Switched |
< 65 ps |
Integration improves
functionality and speed |
Array sources (using
AWG) |
Typically16 channels
spaced by 200 GHz |
100200 ms |
Locked wavelengths and
temperature tuning of whole comb by 275 GHz demonstrated |
Array sources (DFB) |
Limited by number of
elements in array |
110 ns |
DFB lasers act
independently, and can drift and cause crosstalk |
Table 1.
Properties of tunable sources
Erbium-doped
fiber amplifiers (EDFAs) provide optical amplification to compensate
power loss in optical signal transmission and processing (splitting,
multiplexing etc.). Conventional C-band EDFAs cover the 1530–1565 nm
wavelength range and extended L-band. EDFAs cover the 1565–1605 nm
range for a total available gain bandwidth of 9 THz. Commercial
systems have already capitalized on this bandwidth by using 100 GHz
and 50 GHz spaced channels at channel bit rates of up to 10 Gb/s and
a total fiber capacity of greater than 1 Tb/s. Future trends are
toward smaller channel spacing, higher bit rate per channel, and
higher spectral efficiency (bits per second per hertz).
WDM filters are
another key technology; they allow splitting and combining of the
available wavelength band into more than 100 individual wavelength
channels. A variety of WDM filter types are available, such as fiber
Bragg grating filters, dielectric thin-film filters, and Mach-Zehnder
and waveguide-grating-router devices.
Semiconductor
lasers are used in transmitters for the WDM multi-channel systems.
Fixed-wavelength distributed feedback (DFB) semiconductor lasers
with wavelengths on an internationally standardized wavelength grid
are now widely available. Semiconductor lasers tunable over as much
as 40 nm (4.6 THz) are also becoming commercially available.
Filter category |
Tuning range |
Tuning time |
Comments |
Fabry-Perot |
500 nm |
110 ms |
Fiber implementation
available |
Acousto-optic |
250 nm |
10 s |
Can be used as a
router as well |
AWG tunable filter |
40 nm |
10 ms |
Thermo-optic tuning |
Liquid crystal Fabry-Perot |
30 nm |
0.510 s |
Low power consumption
(< 1mW) |
Electro-optic |
16 nm |
110 ns |
Resolvable channels
about 10 |
Fiber Bragg grating |
10 nm |
110 ms |
Temperature or
mechanical stretching tuning |
Cascaded Mach-Zehnder
interferometer filters |
4 nm |
50 ns |
Insertion loss can be
an issue |
Tunable filters based
on semiconduct or laser structures |
5 nm |
0.11 ns |
Small number of
channels |
Table 2.
Characteristics of tunable filters.
Electro-mechanical, and in the very near future
micro-electromechanical system (MEMS), optical fiber switches switch
full-band optical signals between multiple fibers; switching times
are now typically between a few and tens of milliseconds.
Signal splitters,
combiners, and variable attenuators allow further manipulation of
optical signals.
High-speed,
greater than 10 Gb/s, single-channel transmission is enabled by a
variety of optical and electronic components. Among the key devices
here are low-wavelength-chirp external lithium-niobate modulators,
as well as integrated or copackaged semiconductor electroabsorption
and Mach-Zehnder modulators, with modulation bandwidths in excess of
10 GHz.
Commercial PIN
photodetectors and avalanche photodetectors (APDs) provide receiver
bit rates of 10 Gb/s and higher; EDFA-based optical preamplifiers
enable high sensitivity of these optical receivers.
Dispersion-compensating fiber allows compensation of the fiber link
dispersion for high-speed long-distance signal propagation, as well
as tailoring of the dispersion profile in the dispersion-managed
optical signal path.
These and other
optical technologies are being further developed to provide richer
functionality for WDM applications while improving their performance
and reducing component-induced signal impairments.
About TACS
TACS
Consulting Delivers The Insight and Vision on Information
Communication and Energy Technologies for Strategic Decisions.
TACS is Pioneer and Innovator of many Communication Signal
Processors, Optical Modems, Optimum or Robust Multi-User or
Single-User MIMO Packet Radio Modems, 1G Modems, 2G Modems, 3G
Modems, 4G Modems, 5G Modems, 6G Modems, Satellite Modems, PSTN
Modems, Cable Modems, PLC Modems, IoT Modems and more..
TACS consultants conducted fundamental scientific research in
the field of communications and are the pioneer and first
inventors of PLC MODEMS, Optimum or Robust Multi-User or
Single-User MIMO fixed or mobile packet radio structures in the
world.
TACS is a leading top consultancy in the field of Information,
Communication and Energy Technologies (ICET). The heart of our
Consulting spectrum comprises strategic, organizational, and
technology-intensive tasks that arise from the use of new
information and telecommunications technologies. TACS Consulting
offers Strategic Planning, Information, Communications and
Energy Technology Standards and Architecture Assessment, Systems
Engineering, Planning, and Resource Optimization.
|
TACS is a leading top consultancy in the field of information, communication
and energy technologies (ICET).
The heart of our consulting spectrum comprises strategic,
organizational, and technology-intensive tasks that arise from the use of new
information, communication and energy technologies. The major emphasis in our work is found in innovative consulting and
implementation solutions which result from the use of modern information,
communication and energy technologies.
TACS
- Delivers the insight and vision
on technology for strategic decisions
- Drives
innovations forward as part of our service offerings to customers
worldwide
- Conceives
integral solutions on the basis of our integrated business and technological
competence in the ICET sector
- Assesses technologies and standards and develops
architectures for fixed or mobile, wired or wireless communications systems
and networks
- Provides
the energy and experience of world-wide leading innovators and experts in their fields for local,
national or large-scale international projects.
|
WDM Links
WDM technology is
based on the ability to transmit several light signals on a single
fiber using different wavelengths. It turns out that such different
light wavelengths do not interfere with each other, and thus can be
split apart at the other end of the fiber to form separate channels
(Fig.4 is a schematic drawing of such a system). In this figure only
half-duplex channels are depicted. To realize the other direction, a
duplicate system is typically used in that direction The WDM link
essentially comprises the following elements (scanning the figure
from left to right):
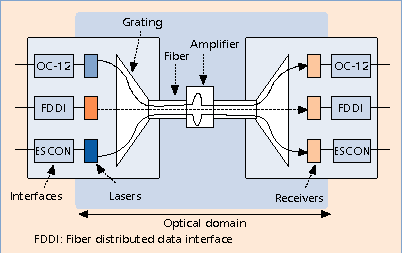
Figure 4 - A WDM
link.
-
·
Different interfaces
per port to enable different protocols to communicate over the
link.
-
·
An electro-optical
converter which includes a laser per channel at different
wavelengths.
-
·
An optical
multiplexer, typically a piece of glass called a grating.
-
·
Due to attenuation,
amplifiers may be needed along the fiber or at the endpoints.
-
·
When the signal gets
to the other end of the fiber it is split by an optical
demultiplexer, which acts like a prism, to separate
wavelength-specific optical signals (the same grating could be
used again).
-
·
A
wavelength-insensitive receiver converts the signal into
electrical form.
-
·
The signal is output
via the specific interface of the channel's port.
The pace of
improvement for this technology is spectacular: while in 1994 the
only commercial product for the telco market multiplexed four OC-48
(2.5 Gb/s) channels to a distance of 550 km, the high-end
multiplexer in 1995 has multiplexed 8 such channels, 16 channels to
600 km in 1996, and a 32-channel system to 1200 km in 1997, and much
more (100s) has been announced currently. As for the enterprise
market, a multi-protocol lower-speed and shorter-distance system has
existed since 1994. As mentioned earlier, such systems are already
deployed and are rapidly gaining popularity due to their maturity,
the large gain they offer, and the simplicity of integration with
legacy equipment.
Several other demonstrations of
WDM that have been reported recently are:
-
·
34 wavelengths x10 Gb/s signal transmitted over 8 514 km fiber using an
equalization technique via Fourier synthesis of the gain
characteristic of the system;
-
·
50 GHz spaced, 32 wavelengths x10 Gb/s signal trans-mitted over 640 km
dispersion-shifted fiber with multi-wavelength distributed Raman
amplification;
-
·
25 wavelengths x40 Gb/s, 1 Tb/s throughput experiment demonstrated over
342 km in nonzero dispersion fiber.
Early deployment
of WDM has mainly been point-to-point to increase link bandwidth,
supports little in terms of networking functionality, and does not
yet perform traffic protection or restoration. In cases of fiber
cuts or network failures, synchronous optical network/synchronous
digital hierarchy (SONET/SDH) equipment would usually provide these
functions, with WDM used strictly for fiber capacity expansion.
WDM Link Enabling Technologies
The optical WDM
revolution in transmission and emerging applications in optical
networking are enabled by a range of key optical technologies. At
the foundation is the low loss, 0.25 dB/km, single-mode optical
fiber, which allows long-distance transmission with a bandwidth
window of about 25 THz.
Source category |
Tuning range |
Tuning time |
Comments |
Mechanically tuned
lasers |
Full range of laser
(1020 nm) |
100500 ms |
Mechanical tuning of
the facets of an FP external cavity |
Acousto-optically and
electro-optically tuned lasers |
Full range of laser
(1020 nm) |
Tens of s |
Find application in
packet switching |
Injection current
tuned lasers |
4 nm |
0.510 ns |
Limited tuning range |
Switched sources |
Switched |
< 65 ps |
Integration improves
functionality and speed |
Array sources (using
AWG) |
Typically16 channels
spaced by 200 GHz |
100200 ms |
Locked wavelengths and
temperature tuning of whole comb by 275 GHz demonstrated |
Array sources (DFB) |
Limited by number of
elements in array |
110 ns |
DFB lasers act
independently, and can drift and cause crosstalk |
Table 1.
Properties of tunable sources
Erbium-doped
fiber amplifiers (EDFAs) provide optical amplification to compensate
power loss in optical signal transmission and processing (splitting,
multiplexing etc.). Conventional C-band EDFAs cover the 1530–1565 nm
wavelength range and extended L-band. EDFAs cover the 1565–1605 nm
range for a total available gain bandwidth of 9 THz. Commercial
systems have already capitalized on this bandwidth by using 100 GHz
and 50 GHz spaced channels at channel bit rates of up to 10 Gb/s and
a total fiber capacity of greater than 1 Tb/s. Future trends are
toward smaller channel spacing, higher bit rate per channel, and
higher spectral efficiency (bits per second per hertz).
WDM filters are
another key technology; they allow splitting and combining of the
available wavelength band into more than 100 individual wavelength
channels. A variety of WDM filter types are available, such as fiber
Bragg grating filters, dielectric thin-film filters, and Mach-Zehnder
and waveguide-grating-router devices.
Semiconductor
lasers are used in transmitters for the WDM multi-channel systems.
Fixed-wavelength distributed feedback (DFB) semiconductor lasers
with wavelengths on an internationally standardized wavelength grid
are now widely available. Semiconductor lasers tunable over as much
as 40 nm (4.6 THz) are also becoming commercially available.
Filter category |
Tuning range |
Tuning time |
Comments |
Fabry-Perot |
500 nm |
110 ms |
Fiber implementation
available |
Acousto-optic |
250 nm |
10 s |
Can be used as a
router as well |
AWG tunable filter |
40 nm |
10 ms |
Thermo-optic tuning |
Liquid crystal Fabry-Perot |
30 nm |
0.510 s |
Low power consumption
(< 1mW) |
Electro-optic |
16 nm |
110 ns |
Resolvable channels
about 10 |
Fiber Bragg grating |
10 nm |
110 ms |
Temperature or
mechanical stretching tuning |
Cascaded Mach-Zehnder
interferometer filters |
4 nm |
50 ns |
Insertion loss can be
an issue |
Tunable filters based
on semiconduct or laser structures |
5 nm |
0.11 ns |
Small number of
channels |
Table 2.
Characteristics of tunable filters.
Electro-mechanical, and in the very near future
micro-electromechanical system (MEMS), optical fiber switches switch
full-band optical signals between multiple fibers; switching times
are now typically between a few and tens of milliseconds.
Signal splitters,
combiners, and variable attenuators allow further manipulation of
optical signals.
High-speed,
greater than 10 Gb/s, single-channel transmission is enabled by a
variety of optical and electronic components. Among the key devices
here are low-wavelength-chirp external lithium-niobate modulators,
as well as integrated or copackaged semiconductor electroabsorption
and Mach-Zehnder modulators, with modulation bandwidths in excess of
10 GHz.
Commercial PIN
photodetectors and avalanche photodetectors (APDs) provide receiver
bit rates of 10 Gb/s and higher; EDFA-based optical preamplifiers
enable high sensitivity of these optical receivers.
Dispersion-compensating fiber allows compensation of the fiber link
dispersion for high-speed long-distance signal propagation, as well
as tailoring of the dispersion profile in the dispersion-managed
optical signal path.
These and other
optical technologies are being further developed to provide richer
functionality for WDM applications while improving their performance
and reducing component-induced signal impairments.
|