|
Wavelength Routing Networks
A scalable
optical network can be constructed by taking several WDM links and
connecting them at a node by a switching subsystem. Using such nodes
(also called wavelength routers) interconnected by fibers, diverse
networks with complex and large topologies can be devised (Fig. 3).
Each wavelength
router makes its routing decision based on the input port and
wavelength of a connection going through it. Thus, if a light signal
enters a router at a port x it is switched to some output port y. At
the other end of the fiber, attached to y, the signal enters another
router in which a similar routing decision is made. This process
continues until the signal is switched to an output port of the
system (Fig.1). Another optical signal coming into the same router
on a different wavelength will be routed differently. Such an
end-to-end connection is called a lightpath, and it provides a
high-speed transparent pipe to its end users. At the same time,
another lightpath can reuse the same wavelength in some other part
of the network, as long as both lightpaths do not use it on the same
fiber. Since such "spatial reuse" of wavelengths is supported by
wavelength routing networks, they are much more scalable than
broadcast-and-select networks.
Another important
characteristic which enables these networks to span long distances
is that the energy invested in a lightpath is not split to
irrelevant destinations. There is a large diversity of capabilities
that a wavelength router can provide, depending on the components in
use and design of the node. Most notably, nodes may provide
· configurable lightpaths versus
· fixed routing,
· full wavelength conversion versus
· limited conversion versus
· no conversion at all,
· fault tolerance in the optical layer versus
· reliance on higher layers.
Nodes also vary
in their
· scalability to
increasing numbers of local or network ports.
Node design options
As for the design
of the node itself, current commercial technology enables either of
the first two of the following designs (Fig.6). The third design
relies on large optical switches and wavelength converters, a
technology far from commercially available and therefore a
longer-term option:
· Electro-Optical
Node
· Simple All-Optical Node
· Full-Conversion All-Optical Node
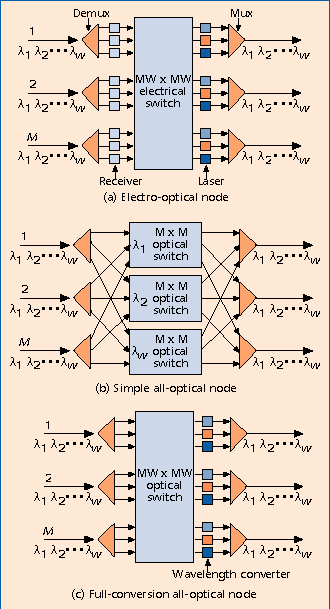
Figure 6 - Three
designs for a wavelength routing node
Electro-Optical Node
Converts the
optical signal into the electrical domain, performs the switching in
this domain, and regenerates the optical signal at the outputs (Fig
6a). This design easily enables wavelength conversion and maintains
a high-quality signal for multiple hops. On the other hand, it does
not support transparency. This design represents an evolutionary
phase toward all-optical networks.
Simple All-Optical Node
Separates the
different wavelengths from each input and sends all channels of
i to the same switch, which optically switches them to the
output ports (Fig. 6b). This design does not allow wavelength
conversion, thereby restricting the reuse of wavelengths in the
system. This may prove to be a cost-effective solution because it
does not require a (costly) transceiver per channel per node.
Full-Conversion All-Optical Node
Enables each
wavelength to be converted to any other wavelength. It is based on a
large optical switch which takes a channel and switches it to any
other channel (on any fiber). Before being multiplexed into the
fiber, each channel is converted to the appropriate wavelength by
fixed wavelength converters (Fig.6c).
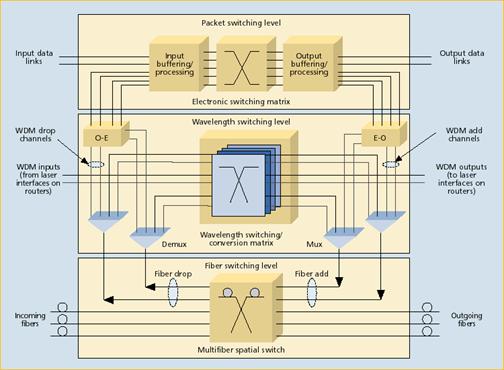
Figure 7 - The
architecture of a hybrid fiber-wavelength-packet (FWP) switching
node of the OTN.
Enabling Wavelength Routing
Technologies
To enable
wavelength routing networks, the maturity of the device technology
needed to manufacture tunable and switched sources, tunable filters,
wavelength converters, wavelength routers, and switching elements is
a key issue. Most recent reported analyses indicate that, for
instance, some of these have a high maturity and can easily be
inserted in real systems (e.g., tunable filters); some others are
still not mature enough for employment in practical systems (e.g.,
wavelength converters). However, we can expect that in the following
two or three years most of them will gain a technological maturity
which will allow the implementation of more complex WDM systems.
Most WDM network
architectures presented so far are based on static or semi-permanent
wavelength routing. This means that the status of the network and
its devices changes very slowly with time, on the order of hours or
days.
Nowadays,
advanced network architectures (intelligent) are hypothesized, which
will allow more flexible and dynamic use of wavelength resources,
depending on the variation of traffic dynamics (Fig.7). This is
particularly true in the case of optical networks for data traffic
(e.g. optical Internet, or optical networks which interconnect
several IP routers).
A relevant
example is represented by multi-protocol lambda-switched optical
networks, which are optical networks compatible with the
multi-protocol label switching scheme proposed for Internet routing.
In this case, dynamic routing in a timeframe of seconds or even less
is required. WDM networks which employ such dynamic and flexible
routing schemes need wavelength agility, that is, the property of
optical devices to rapidly change their working conditions.
Wavelength agile
devices have already been demonstrated. In particular, burst mode
operating receivers and agile wavelength converters have been
realized.
The wavelength
agility characteristic requires technological efforts to render such
devices reliable and well performing. This means that much effort
should be made to push the maturity of the technology at a
reasonable level. Wavelength agility is the key function for
realizing wavelength/time-division multiple access (WDMA/TDMA)
access systems, which require fast tunable transmitters and
receivers to set up individual customer connections through a single
wavelength router (suitably replicated for resilience). In such
networks connection among users is realized by a double dimension
resource: wavelength and time slots.
A further step is
represented by the realization of devices suitable for optical
packet switching (OPS). In this case it is much more difficult to
foresee when and if the maturity of these devices will be such that
OPS networks can practically be realized.
About TACS
TACS
Consulting Delivers The Insight and Vision on Information
Communication and Energy Technologies for Strategic Decisions.
TACS is Pioneer and Innovator of many Communication Signal
Processors, Optical Modems, Optimum or Robust Multi-User or
Single-User MIMO Packet Radio Modems, 1G Modems, 2G Modems, 3G
Modems, 4G Modems, 5G Modems, 6G Modems, Satellite Modems, PSTN
Modems, Cable Modems, PLC Modems, IoT Modems and more..
TACS consultants conducted fundamental scientific research in
the field of communications and are the pioneer and first
inventors of PLC MODEMS, Optimum or Robust Multi-User or
Single-User MIMO fixed or mobile packet radio structures in the
world.
TACS is a leading top consultancy in the field of Information,
Communication and Energy Technologies (ICET). The heart of our
Consulting spectrum comprises strategic, organizational, and
technology-intensive tasks that arise from the use of new
information and telecommunications technologies. TACS Consulting
offers Strategic Planning, Information, Communications and
Energy Technology Standards and Architecture Assessment, Systems
Engineering, Planning, and Resource Optimization.
|
|
|